Advances in Quantum Sensing and Timing
Atomic physicists at the Air Force Research Laboratory (AFRL) are conducting research and development (R&D) of next-generation quantum atomic clocks, quantum sensors and component technologies for the U.S. Space Force and the greater military. The work is necessary to create better clocks, high-performance sensors and related technologies that are more resilient in the space domain. The laboratory, which is spearheading many groundbreaking quantum-related research efforts across the country, classifies quantum R&D into four areas: timing, sensing, computing and networking. Two areas—timing and sensing—are considered the most promising in the near term, noted AFRL’s John Elgin, research physicist and clock and timing lead.
“We are looking specifically into timing, which is [making] better atomic clocks, be it with lower size weight and power (SWAP), meaning that we can provide better performance in a lower SWAP package,” Elgin said. “Therefore, they can be operated in the field easier or [they are] better performing clocks in that they are able to hold time for a longer period of time without the need for updates, referred to as a longer holdover.”
From the sensing side, the AFRL is developing trapped atom or cold atom sensors, with the goal of adding variable dynamic range, Elgin added. “We are [developing] highly sensitive sensors that can sense very small instances all the way up to very large forces and in between. We are also developing component technologies to feed into these low SWAP designs, [such as] miniaturizing frequency cones down to microcones or resonators. And we are working to photonically integrate a lot of these components onto chips so we can integrate those with the physics packages to make these low-SWAP clocks and sensors.”
Regarding the efforts into next-generation atomic clock technology, the AFRL is looking to provide notable improvements over existing space-based timing capabilities, which are critically used as part of global navigation satellite systems and the Global Positioning System (GPS). “Atomic clocks have been around since the ’50s,” Elgin clarified. “We use them in GPS and everything else. They are fundamentally a quantum device in that they exploit the quantum nature of the atom to do the performance and measure the clock and frequency output. But the advantages that you gain from quantum devices that you get over classical sensors, you get dynamic range, which means that you can adjust the sensitivity for very, very small sensing of things to very large things with the same sensor without the need to go to multiple different sensors.”
Elgin earned his doctoral degree at Stony Brook in atomic physics, specializing in laser cooling and tracking techniques and worked as a postdoctoral student at the National Institute of Standards and Technology in Boulder, Colorado. There he helped develop a next-generation, battery-powered, chip-scale atomic clock as part of the Defense Advanced Research Projects Agency Atomic Clock with Enhanced Stability (ACES) program. He partnered with Boulder-based company ColdQuanta to test and provide proof of concept of the ACES clock design. That experience, he said, has proven essential to his efforts at AFRL’s Space Vehicles Directorate at Kirtland Air Force Base in Albuquerque, since he joined the Quantum Sensing and Timing group in 2018 as the timing lead.
“Having an atomic clock background was absolutely necessary,” he said. “The group out here was originally developing an optical atomic clock, the Optical Rubidium Atomic Frequency Standard, or ORAFS.” The inventors included previous AFRL researchers John Burke and Nathan Lemke, along with Gretchen Phelps, Benjamin Stuhl and Kyle Martin, “who were developing a clock design targeted towards the next generation atomic clock for GPS.” ORAFS featured a design built on existing vapor cell and laser technologies. Due to optical power changes, existing GPS clocks have instabilities, and according to the AFRL, “are the most difficult clock instability mechanisms to suppress.” The work on ORAFS, and the research since, has been to overcome the limitations of current space-based clocks.
Another timing-related effort Elgin and scientists at AFRL are working on is time transfer research—being able to share the precision timing from atomic clocks to other clocks. “While we have great atomic clocks and very good, precise time, we need to be able to disseminate that time to adjacent clocks, therefore synchronizing multiple different clocks together,” Elgin said. “We have efforts using two-way optical transfer.”
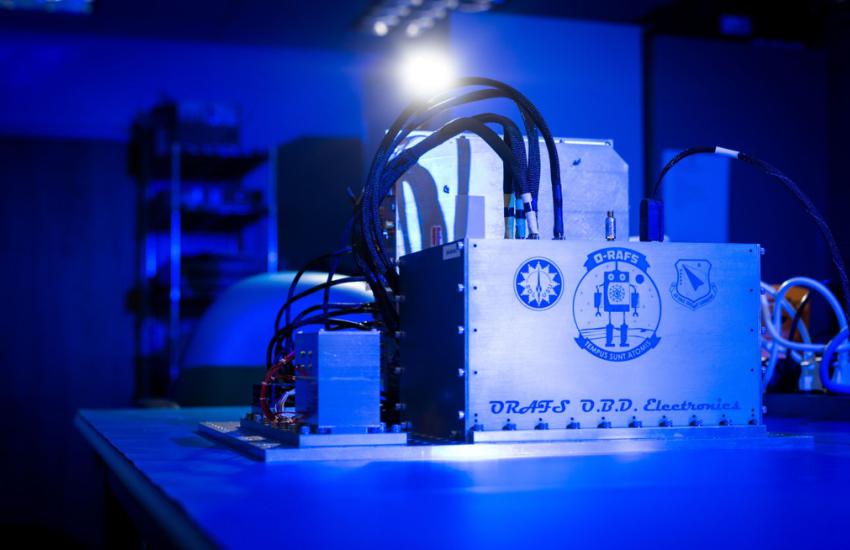
In addition, given the space-based nature of atomic clock use, SWAP improvements are crucial for applications in that domain, and essentially, any SWAP reductions are gained through the component technologies, the timing lead clarified.
“From a quantum physics standpoint, you are working with the physics package, be that your atoms or sometimes it could be trapped ions, but you can only make those so small,” Elgin stated. “What you can do to get lower SWAP beyond that is you can reduce the size of the electronics, integrate the laser and frequency cone into a photonically integrated chip, thereby taking something that is the size of a laptop and reducing it down to the size of a credit card. Our SWAP reductions aren’t necessarily quantum. Quantum is the physics behind it, our SWAP reduction is the engineering components that enable us to take quantum from the lab and put it into the field.”
Meanwhile, the AFRL’s efforts in ultra-cold-atom, quantum-based sensors rest on the ability to trap atoms, which tackles so-called drift concerns and improves timing stability. “In the case of trapped atoms and sensors and systems like this, what we’re actually doing is physically trapping the atoms,” he said. “We keep them in cold, and we isolate them from the environment. [They are] no longer in direct contact with the rest of the sensor, which is no longer in contact with the atmosphere and air, and so we can completely eliminate the drifting concerns in that way. Environmental drifts play a big concern, and quantum allows you to help eliminate that environmental drift. Sensors can now operate and behave long term, without the worry that you’re no longer making the right sensing if you go for long periods of time without an update.”
By leveraging the physics of the contained atoms, the AFRL scientists have found ways to dynamically change the performance of the sensors. “With the trapped atom sensors that we have, the sensitivity of the atoms is controlled by how well we trap the atoms,” Elgin reasoned. “Usually, the way we control the tightness of the trap is by adjusting the optical power or laser power that we put on the atoms. So, if we very loosely trap the atoms, they will move around more, and they’re going to be much more sensitive to very small perturbations in the environment around them and, therefore, will be very sensitive sensors. If we make it very tight, then small perturbations will not be detected, but very large ones will. This allows us to dynamically change our sensitivity and our measurements.”
That discovery will allow sensors to be used depending on desired goals or applications. “If we know we’re very sensitive and interested in something very small, we can set it for a very weak trap and monitor it,” he noted. “And then, if for some reason we do something that results in a very high perturbation, a movement, a shaking or a change of actualization, then we tighten the trap back up and realize that we’re not going to oversaturate the sensors.”
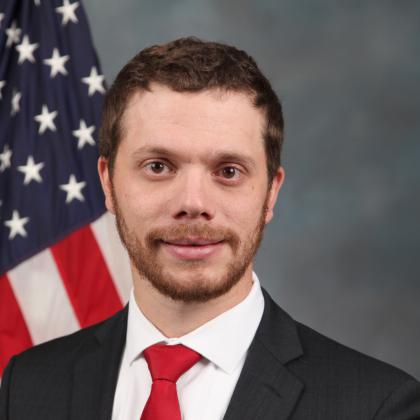
Environmental drifts play a big concern, and quantum allows you to help eliminate that environmental drift,
Moreover, the research on sensors relies on several cold-atom quantum concepts that were awarded a Nobel Prize in physics. “There are three Nobel Prize-winning technologies that we use,” the atomic physicist said. “Two are cold atom, and one of them is more of the optics/laser side of things. The two cold atom capabilities build on each other. In 1997, there was the Nobel Prize for laser cooling, which is the act of actually cooling down atoms through the use of light in interactions, thereby allowing you to cool the atoms to a lower energy state and to trap them into Magneto-optical traps. That subsequently built on to the 2001 Nobel Prize, the Bose-Einstein condensates (BECs). And that is sort of the holy grail of a sensor detector system.”
The AFRL’s research is delving into the BECs to yield breakthroughs, although it is a tough field, Elgin noted. “We haven’t quite crossed that threshold yet due to the complexity of the system,” he said. “But once you get those Bose-Einstein condensates, they now operate as sort of one entity, all the wave functions overlap, and they sort of have a very coherent process, and therefore, they’re much more sensitive to this technology. Our cold atom sensor works in between those stages. It gets beyond traditional Magneto-optical track to a much more tighter track, but it doesn’t quite get all the way to BEC. We have some research efforts looking into easier ways to create a BEC without requiring all the lasers and the complexity that are traditionally done in the normal laboratory environment. But we’re still not to the point where we’re ready to convert that into a full sensor. We know that those sensors have been demonstrated in academia, but a fieldable application for an AFRL site, there is not a direct path there yet.”
Quantum timing and sensor research in the lab requires a lot of equipment and understanding the complexities of the intricate systems, Elgin shared. “You deal with a lot of instances of vacuum chambers and multiple different lasers. And having understanding of your environment very well, be it magnetic fields and electrical interference and concerns with those byproducts so that you understand exactly what state the atoms are in. That results in a lot of vacuum chambers, vacuum pumps, which adds a lot more complexity and size.”
The challenge, after all, is working toward a fieldable system with lower SWAP, he noted. “The vacuum chamber and the lasers make for a very large system,” he stated. “It’s hard, but it is doable. There is sort of a recipe. You could even look up somebody else’s process to create a BEC and optical lattice and all these technologies. You could go buy all the necessary components, and you could put them on the optics table and in about six months to a year, if you had the right students, you could have something operational. The problem is that takes up a full optics table. And it’s not something that you can get out to the field and transport. So, the complexity of the difficulties we have is, ‘How do we lower that, how do we create a lower SWAP system, and how do we create something that’s actually useful and practical for the application sets?’”