The Evolving World of All-Weather Laser SATCOM
Communications using a light beam isn’t a new idea, even outside of “Star Trek,” “Star Wars” and other similar tales of fantasy. Scientist and science fiction writer Arthur Clarke forecasted communications over light beams when the modern age of satellite communications was little more than a dream. SIGNAL Magazine published an article in 1975 featuring laser communications, or lasercomm, devices. Demonstrations of optical communications technologies occurred in the mid-’90s. The Communications Research Laboratory in Japan, for example, successfully demonstrated the Laser Communication Experiment on the Japanese Engineering Test Satellite-VI in 1994, which was the first purpose-built lasercomm satellite to illustrate space-to-ground laser communications.
The reason for this interest in lasercomm is that optical communication systems, as we know them today, have several advantages over the currently used UHF, SHF and EHF systems, including higher data rates (so more information may be transmitted in less time and using lower power), better signal/noise ratios (weather-dependent) due to higher directivity, a lack of interference, smaller antennae, lower overall power requirements, increased spectrum availability, narrow beams that are more difficult to intercept and jam, and no International Telecommunication Union coordination needed to establish networks.
As noted, a principal advantage exists with capacity. The light spectrum is several thousand times larger than the radio frequency (RF) spectrum; thus, while the RF goes from approximately 300 hertz to 300 gigahertz, the light spectrum runs from around 400 to 800 terahertz. The frequencies are so high and require so many zeros that lightwave communications systems are referred to in nanometers (nm), 800 nm being a typical wavelength/frequency. While the achieved data rates depend on the signal-encoding scheme (among other things), in general, they could be up to a thousand times higher than rates in RF communication.
Satellite lasercomm has been a feature of the Department of Defense (DoD) planning for a number of years. Those who worked on the ill-fated Transformational Satellite Communications Program (TSAT) saw the essential need to connect TSAT’s orbital laser satellite net with the Defense Information Systems Network’s worldwide fiber network, which connects the orbital laser ring in space to the terrestrial worldwide laser ring of worldwide fiber. The solution was to deploy earth stations to geographically dispersed, moderate weather locations, thus avoiding the dissipating effects of rain, drizzle, clouds, fog and dust.
And that solution illustrates the disadvantages of optical communications systems as they are known today. These systems have a higher pointing accuracy needed for satellites, increased complexity and availability risk, a noise source for optical receivers in the sun, and as noted, in rain, drizzle, clouds, fog and dust, the major disrupters.
Despite the atmospheric handicap, several experiments and systems are using space-to-Earth lasers. NASA’s Laser Communications Relay Demonstration (LCRD) has demonstrated two-way laser communications from geosynchronous orbit since early 2022.
The drawing boards, brass boards, prototypes and initial launches of the megasatellite constellations feature multiple laser-dependent networks. Canada’s Telesat, with its Lightspeed constellation, possibly the epitome of a laser communications network, is developing satellite-to-satellite connectivity in similar and dissimilar orbits. Although the system has been beset with financial difficulties, design changes and increased investment appear to be putting it back on track. SpaceX’s path-forging Starlink satellite internet service, which has launched over 5,000 satellites, confirmed using laser satellites to provide internet connectivity to several regions last year, although that was also space-to-space only. There are more than 25 systems and concepts for low-Earth-orbit (LEO) satellites giving laser terminal manufacturers an abundance of requests for proposals and contracts.
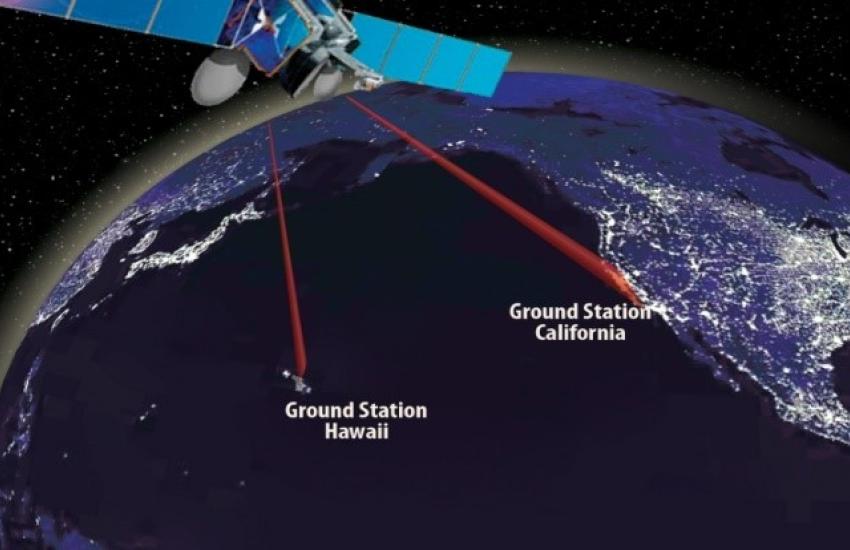
Across the pond, Artemis, the European Space Agency Advanced Relay and Technology Mission Satellite, has been experimenting with laser satellite communication since the early 2000s. More recently, they demonstrated bidirectional laser communication between a geostationary orbit and the European Space Agency Optical Ground Station. In the United States, the Massachusetts Institute of Technology Lincoln Laboratory’s Lunar Laser Communication Demonstration, consisting of a small space terminal and a ground terminal, demonstrated laser’s capacity for extremely high data rates. The space terminal’s three small modules, the optical module or telescope, the modem and the controller, made up a compact, lightweight, power-efficient unit with this NASA-sponsored experiment. The LCRD, creates a continuous path for data flowing from missions in space to ground stations on Earth. Capable of simultaneously sending and receiving data from missions and ground stations, LCRD is NASA’s first two-way, end-to-end optical relay system.
Last year the Space Development Agency awarded two prototype agreements with a total potential value of more than $1.3 billion for the establishment of the Tranche 1 Tracking Layer, providing the initial missile warning/missile tracking warfighting capability of the National Defense Space Architecture. These satellites are scheduled to launch in April 2025. The agreements were awarded to teams led by L3Harris Technologies and Northrop Grumman Strategic Space Systems to each build a space segment consisting of two planes with seven space vehicles per plane–14 satellites from each performer for a total of 28 satellites in four planes—to collect infrared data and provide network communications. Each agreement includes a launch services segment for launch preparation and a ground segment for operations and sustainment. Earlier this year, Raytheon Technologies was awarded the seven-vehicle mission satellite constellation. Each satellite will feature three optical communications terminals and a Ka-band, multibeam payload for communications. For the Earth segment, Mynaric will supply 21 CONDOR Mk3 terminals to Raytheon for the program, with product deliveries expected in 2024.
The initiatives are, of course, not just U.S. and European. Space War noted in July the Aerospace Information Research Institute under the Chinese Academy of Sciences, in partnership with the Chang Guang Satellite Technology, successfully conducted a satellite-to-ground laser communication experiment at a speed of 10 gigabytes per second using a self-developed 500-millimeter-diameter ground system and Jilin-1 satellite. The experiment reportedly obtained good-quality satellite payload data, which will meet the requirements of high-standard commercial applications. This experiment demonstrated that China has successfully realized the engineering application of satellite-ground laser high-speed communication. The bottom line is that laser communications through space, or free space optical as it is now frequently called, is growing rapidly to meet the widespread wireless demand of networked high-capacity satellites.
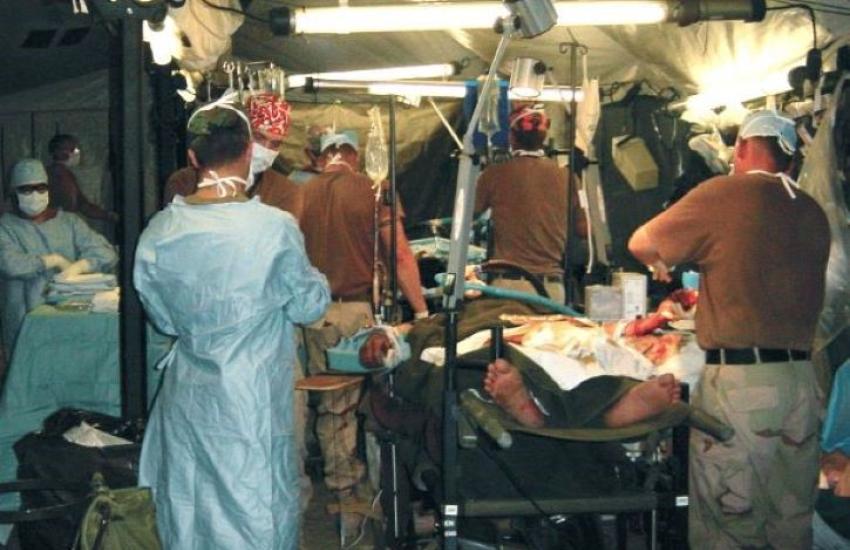
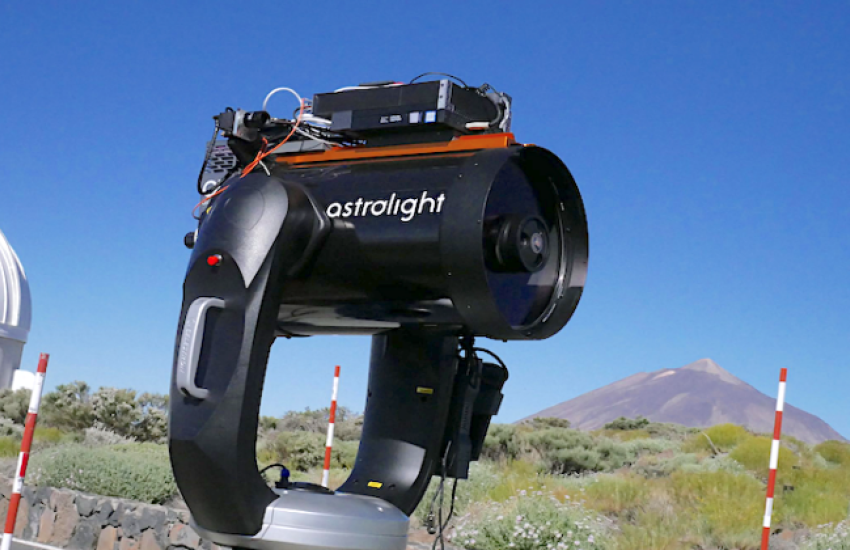
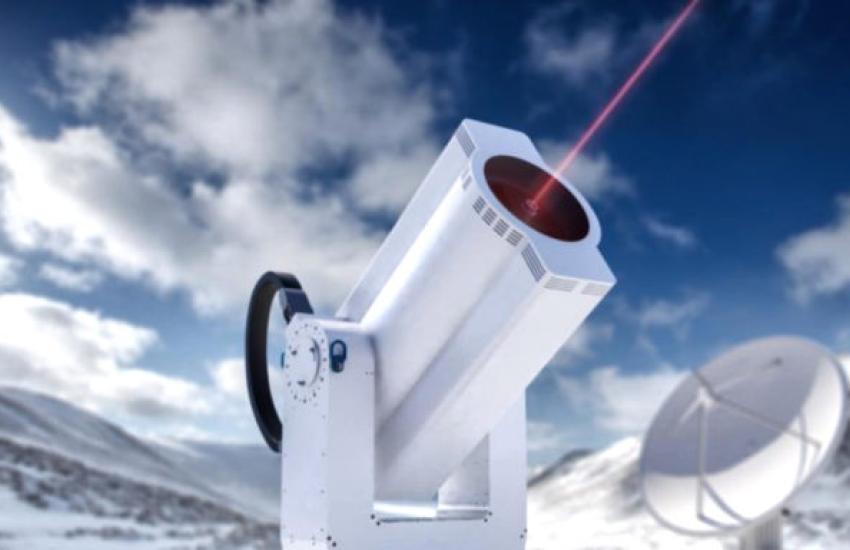
So, when one looks at TSAT and the NASA experiments, the dependency on clear weather appears to be fundamental. The essential reality is that the randomness in distribution and size of the water droplets that make up a rain cloud leads to substantial scattering of the optical energy and rapid scrambling of the information signal encoded in the laser beams. To overcome that scrambling problem, many organizations around the world have looked at methods of penetrating clouds, rain and dust. Theoretically, very high power will work. But that, of course, requires an uneconomical and possibly dangerous amount of power. The last decade has seen a plethora of activity looking at penetration of these atmospherics. High-power continuous-wave CO2 lasers have been successfully used to improve line-of-sight visibility, but as noted, the intensity requirement makes the configuration impractical.
However, it turns out that multiple filaments can be generated by structured light that creates two rotating beams, one stronger and occupying greater angular space. That beam becomes a fiber-like waveguide through space and air. Then, the signal-carrying laser can be guided in through that waveguide, unobstructed by clouds, drizzle or rain. So, the outside ring makes the path, and the signal-bearing laser slowly passes through what has become its protecting channel. This technological approach is being investigated at some length in academic laboratories in Switzerland, France and universities (Rensselaer Polytechnic Institute and the University of Maryland) in the United States. Fortunately, these innovational approaches align with the DoD’s program needs of multiple free space optical initiatives, bringing a high-impact opportunity that could have applications not only in satellite communications but also with subspace relays using atmospheric and stratospheric platforms.
So, what are the implications? Consider a company of soldiers on patrol using a small laser receive system such as the Astrolight terminal shown in the photo at left.
A Global Hawk unmanned aircraft system or even pilotless drone can cover the entire area, feed the information via a satellite system back to a ground entry point, which then distributes it to multiple areas of command, showing not a photograph but an effectively same-time video of the unit and the area surrounding that encampment. Consider also forward surgical care where physicians on the battlefield can have laser link communications with the specialists at the Uniform Services University so that wounds and diseases can be analyzed with as much clarity as if the experts in metropolitan Washington, D.C., were in the operating tent.
Clarity and detail for military operations will soon be available at an unprecedented level, making “the net” an even more significant battlefield component.
James A. Mazzei is a satellite communications systems engineer and a former adjunct professor at the George Mason University, Johns Hopkins University and University of Maryland graduate schools. He teaches the Satellite Communications Course for the AFCEA Professional Development Center. He can be reached at jmazzei@edmondsci.com.
The opinions expressed in this article are not to be construed as official or reflecting the views of AFCEA International.